Dive physics is one of life’s most feared things, alongside public speaking and death. We are here to change that. Over the coming weeks, we are releasing a series of articles with the goal of making you an expert on the laws and mechanisms that rule the activity you so love.
This week, we begin first with the fundamental laws governing the physics of the underwater world, those being: Henry’s, Dalton’s, and Boyle’s Laws. We also touch on the basic concepts relating to decompression, that of on-gassing, off-gassing, saturation and supersaturation. After reading this article, you will be well equipped with the basic principles of diving, allowing you to understand higher-level concepts with ease. Hopefully, by the end of our series, dive physics will have transformed from foe to friend.
Boyle’s Law
‘The volume of a gas at constant temperature varies inversely with the pressure exerted on it.’
Simply put, Boyle’s Law explains the inverse relationship between pressure and volume. Namely, Boyle’s Law tells us two things:
- When pressure is increased, volume decreases.
- When volume increases, pressure decreases.
Unlike other physical laws of diving, we encounter Boyle’s Law from our very first immersion. The first instance it is demonstrated to us is in the fact we must equalise our airspaces. As we descend, the ambient pressure increases. Surface pressure is 1 bar, and pressure increases by 0.1 bar per metre. This can be seen in the table below.
DEPTH (METRES) | PRESSURE (BAR) |
0 | 1 |
10 | 2 |
20 | 3 |
30 | 4 |
40 | 5 |
Consequently, the volume of our airspaces decreases as the ambient pressure increases. We must compensate for this by equalising them with the ambient pressure, i.e. allowing gas to flow into our ears and sinuses, as well as maintaining a normal breathing pattern to equalise our lungs. Similarly, the gas in our BCD is compressed as we descend. If we were to not inflate our BCD as we descended, the volume within it would continue to decrease as the ambient pressure increased, causing us to sink deeper and at an increasing rate. Thus, we must add gas to our BCD in order to compensate for Boyle’s Law (i.e. decreasing volume with increased pressure).
Equally, when we ascend, the ambient pressure decreases. This causes the volume to increase. Thus, we must remove gas from our airspaces, in order to avoid shooting to the surface (and the associated risks/injuries that it carries). Therefore, as we ascend we release gas from our BCD. Similarly, this is why it is fatal to hold one’s breath when ascending; as the volume will continue to expand until the lungs burst.
Dalton’s Law
‘The total pressure exerted by a mixture of gases is equal to the sum of the partial pressures of the individual gases.’
Dalton’s Law has a scope reaching beyond the basic level of this article, from oxygen toxicity and gas planning, to decompression considerations. However, what it shows us at the basic level is how partial pressures of individual gases add up to make the total pressure of a mixture of gases, and how this relates to ambient pressure.
The atmosphere is composed of a mixture of gases. Namely: oxygen (21%) and nitrogen (79%). Of course, there are also trace gases, however, these are not relevant to diving and thus we use the percentages as stated. In order to calculate the partial pressure of a given gas, we simply multiply the fraction of gas (i.e. the percentage of a given gas present) by the ambient pressure. For example, if we were to breathe normal air at a depth of 30 metres (4 bar):
- PPN2 = FN2 x PAMBIENT
- PPN2 = 0.79 x 4
- PPN2 = 3.16 bar
We can then calculate the partial pressure of oxygen at this depth, in the exact same way:
- PPO2 = FO2 x PAMBIENT
- PPO2 = 0.21 x 4
- PPO2 = 0.84
We can then add both of these together, which itself gives the ambient pressure at 30 metres (4 bar):
- PPN2 + PPO2 = PAMBIENT
- 3.16 + 0.84 = 4
The simple beauty of Dalton’s Law is best demonstrated in calculations regarding gas planning for technical divers. If you have an upcoming technical course, are an instructor in training or simply a diver who wants to expand their knowledge, then check out our upcoming articles on Narcosis, Oxygen Toxicity and Gas Planning.
Henry’s Law
‘At a constant temperature the amount of gas dissolved in a liquid is directly proportional to the partial pressure of that gas in equilibrium with that liquid.’
Henry’s Law explains why we on-gas, off-gas, and why our bodies are constantly trying to reach saturation. Simply put, Henry’s Law states that if the partial pressure of a gas in contact with a liquid increases, then the amount of gas molecules dissolved in that liquid will increase proportionately. Therefore, the partial pressure of the gas will always be equal to the partial pressure of dissolved gas in the liquid. Henry’s Law can best be explained by using it to answer a fundamental question:
The deeper we dive, the more nitrogen (inert gas) is absorbed by our tissues – why?
As previously established, with increased depth comes increased pressure. Surface pressure is 1 bar, and pressure increases by 0.1 bar per metre. This can be seen in the table below.
DEPTH (METRES) | PRESSURE (BAR) |
0 | 1 |
10 | 2 |
20 | 3 |
30 | 4 |
40 | 5 |
This increased pressure represents increased weight on our lungs. If we were to continue breathing a gas at surface pressure (1 bar) when submerged to a depth of 10 metres (2 bar), for example, our lungs would not be able to inflate themselves as the ambient pressure is too high. In order for our lungs to counteract this increased weight (pressure) when breathing, the gas we breathe must be equal in density to the ambient pressure. The first stage of a scuba regulator is responsible for this. The first stage detects the ambient pressure. It then opens the valve to the degree necessary to supply us with gas that is equal to the ambient pressure. The denser a gas, the more molecules it contains. The more the valve opens, the more gas molecules will flow out. Thus, the deeper we go, the more the valve will open, allowing a greater flow of molecules, thereby resulting in a denser gas being supplied to the diver. This has the effect of negating the increased pressure, as if we were breathing normally as we do on the surface.
However, this increased gas density comes at a cost, as a direct result of Henry’s Law. As stated previously, Henry’s Law means that the partial pressure of dissolved gas in a tissue will always be equal to that of the gas in contact with the tissue. Therefore, when we are at surface pressure (i.e. in everyday life), dissolved gas in our tissues (which we treat as a liquid) has a partial pressure of 1 bar (equal to the gas surrounding it). This is known as being in a state of saturation, in which the gas dissolved in our tissues is equal to the ambient pressure. This is shown below.
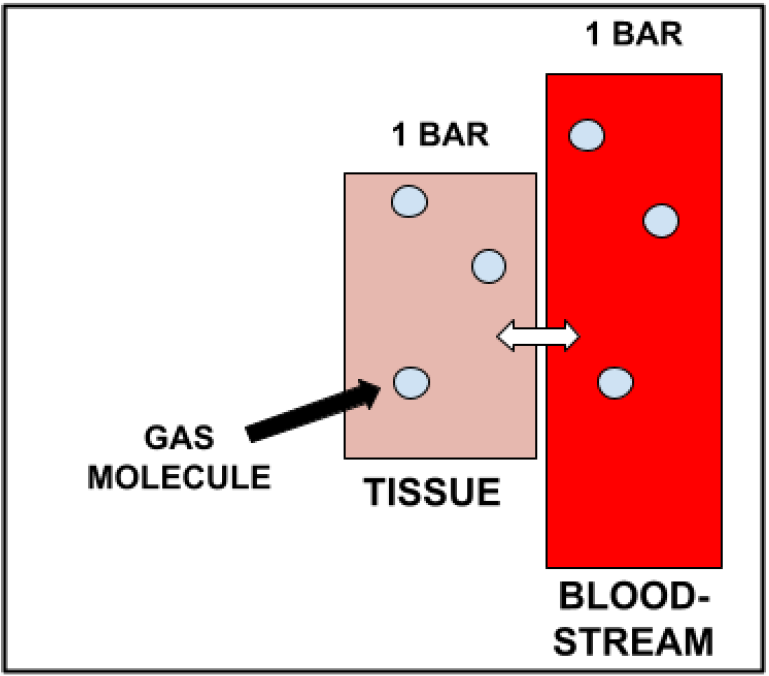
However, as we dive deeper, the gas we breathe becomes denser. This means that there are more total gas molecules per breath, compared to the amount dissolved in our tissues. Thus, the partial pressure of inspired gas will be momentarily higher than that of the dissolved gas in our tissues, resulting in an imbalance/disequilibrium. Due to Henry’s Law, the gas dissolved in our tissues will increase until equal to that of the partial pressure of the inspired gas. This happens by a movement of gas molecules from the higher concentration bloodstream (inspired gas) to the lower concentration tissue. The diagram below demonstrates this process.
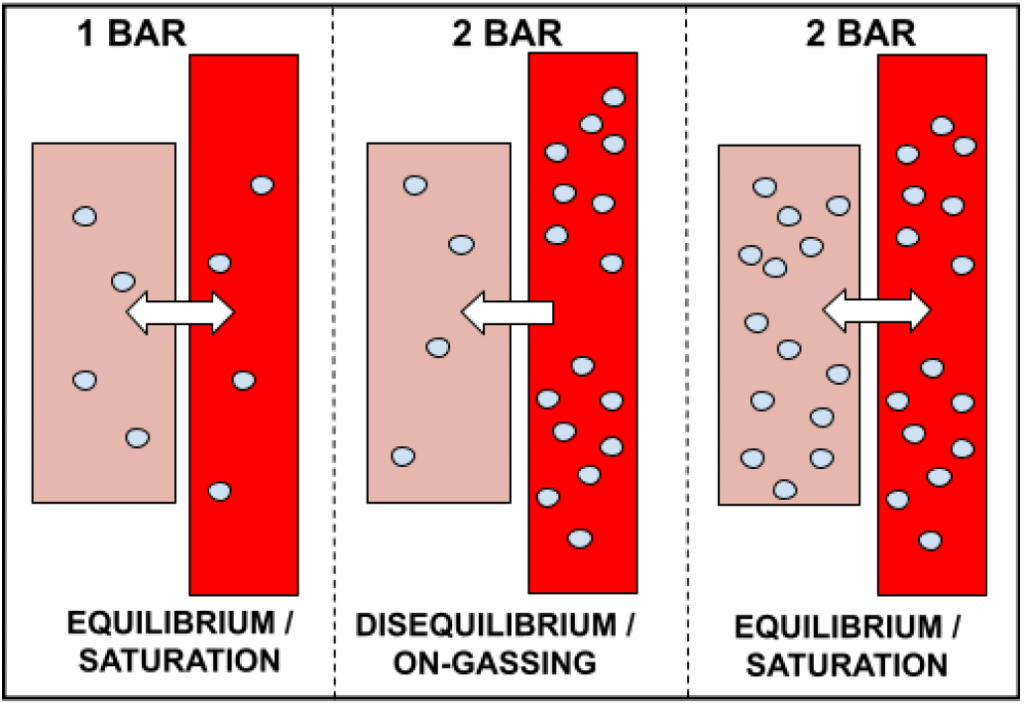
In the example above, the diver dives to 10 metres (2 bar). As they descend to 10 metres, the ambient pressure is increased to 2 bar. Thus, the gas they breathe becomes twice as dense by the regulator supplying twice as many molecules per breath. This means that the inspired partial pressure of gas (specifically nitrogen) is greater than the dissolved partial pressure in the diver’s tissues. Therefore, the gas (nitrogen) molecules will diffuse into the diver’s tissues in an attempt to re-saturate their body with the ambient pressure.
This is known as the process of on-gassing. On-gassing, as the phrase suggests, simply refers to the increase in the amount of dissolved gas in our tissue, or, more specifically, the movement of inert gas into our tissues. This happens everytime we go diving, no matter how shallow the dive may be. On-gassing occurs because our body is constantly trying to achieve a state of equilibrium with the ambient pressure. Therefore, in the example above where the diver dives to 10 metres (2 bar), inspired gas moves from high concentration in the bloodstream, to the less concentrated area in the tissues, and continues to do so until the concentrations are equal to one another (i.e. until the partial pressures are the same). Thus, if the diver stays long enough at 10 metres, saturation will once again be achieved.
So far, we have established the workings of Henry’s Law, and how it explains the concepts of saturation and on-gassing. Lastly, we can now turn to the concepts of supersaturation and off-gassing, again employing Henry’s Law in our explanation.
Supersaturation & Off-Gassing
Saturation describes a state of equilibrium, namely, when the partial pressure of dissolved gas in our tissues is equal to that of inspired gas (i.e. the ambient pressure). On-gassing describes a state of disequilibrium, namely, when the partial pressure of inspired gas is greater than that of dissolved gas in our tissues (thus leading to a movement of gas into our tissues). Off-gassing, therefore, is similar to on-gassing insofar as it also describes a state of disequilibrium. However, it is the reverse disequilibrium: off-gassing occurs when the partial pressure of dissolved gas in our tissues is greater than the partial pressure of the inspired gas. This leads to a movement of gas out of the tissue. This is demonstrated in the diagram below.
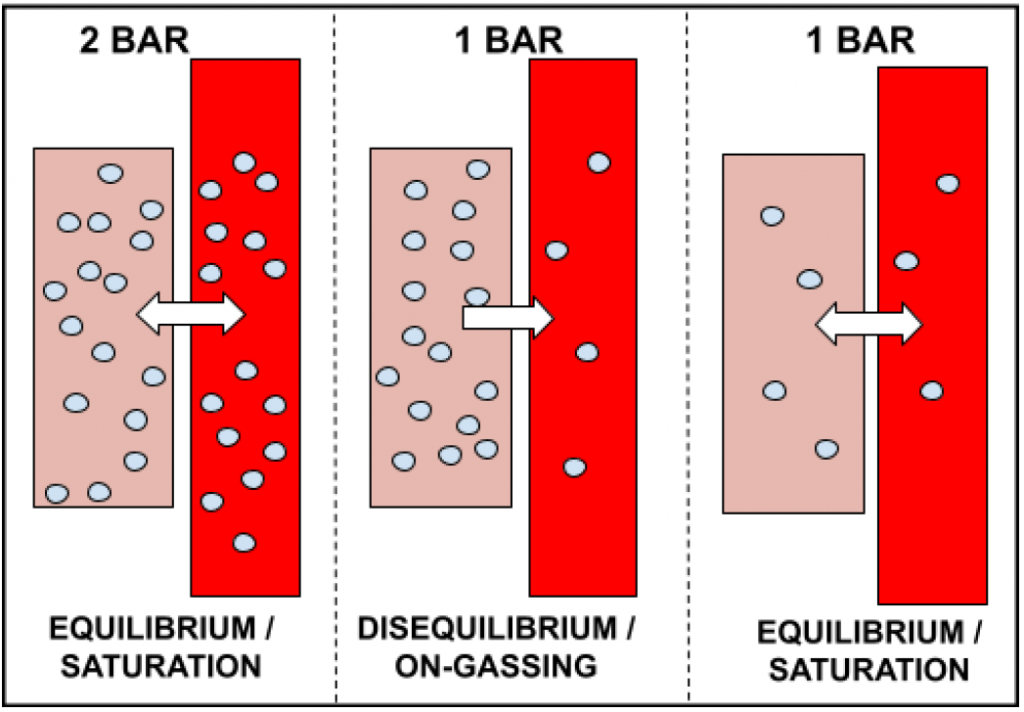
In the example above, the diver ascends from 10 metres (2 bar) back to the surface (1 bar), after having stayed long enough at 10 metres to become saturated. Upon reaching the surface, the partial pressure of dissolved gas in the tissues is greater than the inspired gas pressure, as the ambient pressure has now decreased from 2 bar to 1 bar. Therefore, dissolved gas begins to move out of the tissue, to lower concentrated areas, in an attempt to reach saturation. After having stayed on the surface long enough, the tissues eventually become saturated to 1 bar. This is the phenomenon of off-gassing.
The term supersaturation simply describes that the tissues are ‘over-saturated,’ i.e. have a greater amount of dissolved gas molecules than that in the inspired gas. Just as with on-gassing, off-gassing and supersaturation occur on every dive.
Now that we have established the basics of Henry’s Law, as well as that of Boyle’s and Dalton’s, check out another article in our education series – “Tissues and M-Values” for an in-depth look into supersaturation and how it relates to dive safety and decompression, for both recreational and technical divers. For any Divemasters and/or Instructors in training out there, be sure to check out our Education series, so that you can ace the dive theory portion of your training.